Using EMI and pXRF to Characterize the Magnetic Properties and the Concentration of Metals in Soils Formed over Different Lithologies
Two sites located in the Northern Piedmont of Pennsylvania suspected to have different levels of magnetic susceptibility (k) were examined using electromagnetic induction (EMI) and portable X-ray fluorescence (pXRF). One site is underlain by micaceous schist and serpentinite; the other site by micaceous schist only. The responses of an EM38-MK2-1 meter and the estimated k were greater and more variable at the site underlain by serpentinite and micaceous schist. Also, the average concentrations of Fe, Cr, Ni, and Ti were significantly higher at this site, and significant correlations were derived between the concentrations of several metals and the in-phase response and k of the upper 30 cm of the soil. These correlations were generally lower and less significant at the site underlain by micaceous schist alone. As k is associated with greater amounts of ferromagnetic constituents in soils, the greater concentration of Fe measured with pXRF at the site underlain by micaceous schist and serpentinite helps explain the greater averaged and more variable EMI responses measured with the EM38-MK2-1 meter at this site. The contrast in the EMI and pXRF data between these two sites was associated with differences in the mineralogy and lithologies of serpentinite- and nonserpentinite-derived soils.
Introduction
Little is known about the magnetic properties of soils and their spatial variability, but they are largely determined by the presence of iron oxides in different forms and concentrations [1]. The magnetic susceptibility (k) indicates the presence of iron-bearing minerals in soils and rocks. As k describes a material's ability to become magnetized, it is roughly proportional to the concentration of ferromagnetic minerals. However, the in-phase (IP) response of electromagnetic induction (EMI) sensors is also used to measure the magnetic properties of soils [2].
EMI surveys traditionally focus on the electrical properties of soils, neglecting the magnetic ones. In general, k of most soils is low and has negligible effects on electromagnetic field strengths; however, in magnetic soils, the presence of ferromagnetic minerals interferes with the efficiency of magnetic and electromagnetic sensors in detecting buried metallic objects [1].
The interpretation of k and IP data obtained from EMI sensors remains challenging due to various technical and environmental limitations, especially EMI sensor drift [3], arbitrary "zero level" [4], limited exploration depths [5], and changes in the sign (±) of the response at certain depths and in relation to the target position [6]. Regarding the latter, for EMI sensors operating in the vertical dipole orientation (VDO), the IP response experiences a sign change with depth [2]. For example, for the EM38 meter operating in the VDO, the response is positive for the upper 60 cm of the soil profile and weakly negative below 60 cm [2].
Register now to read the full article as well as all other premium content of Advanced Optical Metrology.
Introduction
Little is known about the magnetic properties of soils and their spatial variability, but they are largely determined by the presence of iron oxides in different forms and concentrations [1]. The magnetic susceptibility (k) indicates the presence of iron-bearing minerals in soils and rocks. As k describes a material's ability to become magnetized, it is roughly proportional to the concentration of ferromagnetic minerals. However, the in-phase (IP) response of electromagnetic induction (EMI) sensors is also used to measure the magnetic properties of soils [2].
EMI surveys traditionally focus on the electrical properties of soils, neglecting the magnetic ones. In general, k of most soils is low and has negligible effects on electromagnetic field strengths; however, in magnetic soils, the presence of ferromagnetic minerals interferes with the efficiency of magnetic and electromagnetic sensors in detecting buried metallic objects [1].
The interpretation of k and IP data obtained from EMI sensors remains challenging due to various technical and environmental limitations, especially EMI sensor drift [3], arbitrary "zero level" [4], limited exploration depths [5], and changes in the sign (±) of the response at certain depths and in relation to the target position [6]. Regarding the latter, for EMI sensors operating in the vertical dipole orientation (VDO), the IP response experiences a sign change with depth [2]. For example, for the EM38 meter operating in the VDO, the response is positive for the upper 60 cm of the soil profile and weakly negative below 60 cm [2].
In the Piedmont of southeastern Pennsylvania and northeastern Maryland, areas of metamorphosed ultramafic rocks, which include serpentinite, occur along the state line [7]. Serpentinite is a Fe- and Mg-rich, subsiliceous rock formed principally through the metamorphic alteration of dunite, peridotite, or pyroxenite [8]. Soils formed over serpentinite have high Mg and low Ca levels, are low in essential nutrients, and have high concentrations of heavy metals [9]. During an EMI soil investigation conducted on a serpentine barren in southeast Pennsylvania, considerable variations in the IP and quadrature-phase (QP) responses were recorded. The cause of these unexpected results was attributed to the k of the soils formed over ultramafic rocks. As a result of these observations, a study was initiated to better understand the EMI response on soils formed over different lithologies in the northern Piedmont of southeastern Pennsylvania and determine whether the concentrations of different metals in these soils could be linked to the response of an EMI meter and k.
Methods
Two study sites, Nottingham Park (39.7375° N, 76.0326° W) and Cochranville (39.8735° N, 75.9315° W), were selected for this study. These sites are located about 12 miles apart in southwestern Chester County, Pennsylvania. The soils on these two sites formed over different lithologies. The Nottingham Park site consists of two portions (Glenelg and Chrome soils) and is located over ultramafic rocks and schist. The Cochranville site consists mainly of Glenelg soil is situated over schist. The full article of this digest includes a detailed description of both sites.
Pedestrian surveys were completed across each site with the EM38-MK2-1 meter (Geonics Limited) operated in the VDO and a continuous recording mode with measurements collected at a rate of 1 s-1. The meter's long axis was orientated parallel to the traverse direction and held about 5 cm above the ground surface. Walking in a back and forth manner across each site along essentially parallel traverse lines, a total of 4465 and 4874 apparent electrical conductivity (ECa) and IP measurements were recorded with the EM38-MK2 meter at the Nottingham Park and Cochranville sites, respectively. The Geonics DAS70 Data Acquisition System was used with the EM38-MK2-1 meter to record and store both EMI and GPS data. At the time of the EMI surveys, soils were moist throughout.
At each study site, a minimum number of soil sampling points were selected by submitting the IP EMI data to the Response Surface Sampling Design (RSSD) program of the ESAP (ECe Sampling, Assessment, and Prediction) software. RSSD was used to statistically select a small number of sample locations based on the observed magnitudes and spatial distribution of the IP EMI data; 12 and 7 optimal sampling points were identified at the Nottingham Park and Cochranville sites, respectively.
Two additional measurements were made at each of the optimal sampling points with the EM38-MK2–1 at different heights (0 and 150 cm). Using these data, k of the soil was estimated following the procedures of Geonics Limited [5]. Small grab samples were collected from the 0 to 30 cm and 30 to 60 cm depth intervals at each sampling point. The samples were dried and analyzed in sampling bags positioned at a constant distance from the portable X-ray fluorescence (pXRF) spectrometer mounted on a portable workstation (Figure 1). A DELTA™ Standard pXRF spectrometer (Olympus®) was used to determine the concentrations of 15 metals (K, Ca, Ti, Cr, Mn, Fe, Co, Cu, Zn, As, Rb, Sr, Zn, Ba, and Pb) in the samples from each site. The spectrometer was calibrated, and each sample was scanned for 60 seconds. For each sample, scans were repeated three times, and an average value was calculated.
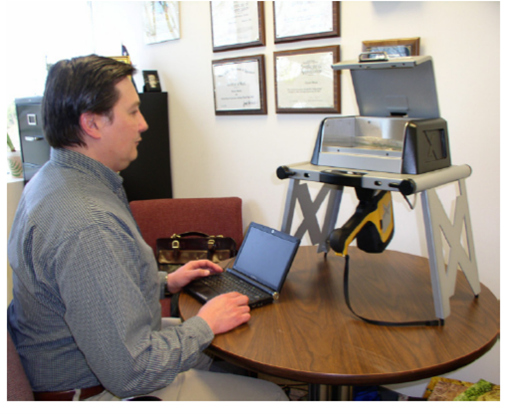
Results and Discussion
Electromagnetic Induction
A statistical summary of the EMI data collected at the Nottingham Park site is shown in Table 1. The ECa averaged 14.3 mS m-1, ranging from 2.6 to 31.3 mS m-1. The comparatively low averaged ECa was assumed to reflect the effects of soil weathering and the relatively shallow depths to bedrock. The IP data averaged 1.8 ppt, with an exceptionally large range of -172 to 359 ppt. The k averaged 9077 × 10-6, ranging from -2850 to 44802 × 10-6. The unusually large ranges in the IP response and k were attributed to the presence of iron-bearing minerals in soils and rocks. Similar variations have been observed in urban areas (e.g., anthropogenic soils) where the induced electric currents are significantly affected by the presence of metallic artifacts (e.g., pipes). No evidence was found of former structures, mining activities, or cultural deposits within the Nottingham Park site.
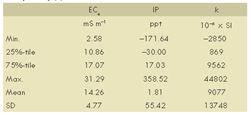
Figure 2 shows the spatial distributions of the ECa and IP data collected at the Nottingham Park site. In each plot, segmented lineations with more extreme and anomalous values can be identified. These lineations suggest layers of contrasting lithology and mineralogy.
In the IP data plot (Figure 2), the higher amplitude (±) anomalies indicate "stronger" source objects. A "stronger" source object may be more conductive or magnetic, larger, and/or located closer to the surface. The amplitudes of the anomalies also depend on the source objects' orientation in the earth's magnetic field. This is especially true for elongate bodies such as veins of highly magnetic materials.
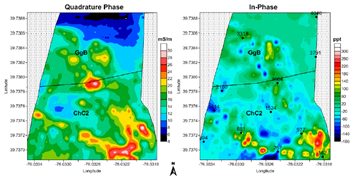
Table 2 lists the basic statistics for the EMI data collected at the Cochranville site. ECa averaged 10.3 mS/m-1, ranging from -0.1 to 15.5 mS m-1. The IP data averaged -24.8 ppt, ranging from -48.0 to 10.1 ppt, and k averaged 1897 × 10-6, ranging from 798 to 5016 × 10-6. ECa/IP measurements and k estimates were noticeably lower in magnitude and less variable at the Cochranville site than at the Nottingham Park site. The contrast in the EMI data between these two sites indicates differences in mineralogy and lithologies.
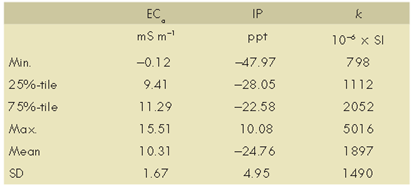
Figure 3 shows the spatial distributions of ECa and IP data at the Cochranville site. Spatial patterns are nondescript except for a noticeable cluster of anomalous values in the extreme north-central portion of the site in the IP data plot. A small outcropping of the Peters Creek schist was evident in the vicinity of this anomalous pattern, and the area was associated with shallower depths to rock and contrasts in mineralogy.
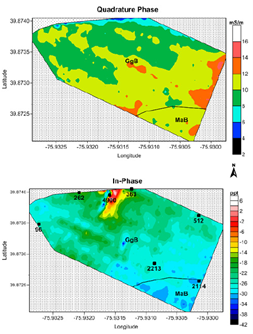
X-Ray Fluorescence
At both sites, the concentrations of different metals varied over several orders of magnitude. Spatial and depth variability in these concentrations was evident at each site. Noticeable differences in the concentrations of each metal were also evident between the sites. The full article of this digest contains tables with the concentration of every measured element in the samples collected at both sites and depth intervals.
At both sites and depth intervals, Fe was the most abundant element. The average concentration of Fe increased with increasing soil depth. However, the average concentration of Fe for the 0- to 60-cm depth interval was about 152% higher at the Nottingham Park site than at the Cochranville site. At both sites, the concentration of Ca decreased with increasing soil depth. At the Nottingham Park site, the mapped Chrome soil is known for its low Ca/Mg ratios [10]. However, the average concentration of Ca for the 0- to 60-cm depth interval was about 188% higher at the Nottingham Park site than at the Cochranville site.
The Chrome soil, which forms over serpentinite, typically has high Ni and Cr concentrations [9,10]. The average concentration of Cr for the 0- to 60-cm depth interval was about 11 times higher at the Nottingham Park site than at the Cochranville site. Although less abundant, the average concentration of Ni was markedly higher at the Nottingham Park site than at the Cochranville site (960 and <20 mg kg-1, respectively).
Correlation between EMI and P-XRF Data
Because of the small number of soil samples obtained from each site, nonparametric statistics were used to access the relationship between EMI and pXRF data. Table 3 summarizes the correlations between EMI and pXRF data for both depth intervals at the Nottingham Park site (only the eight most abundant metals are listed).
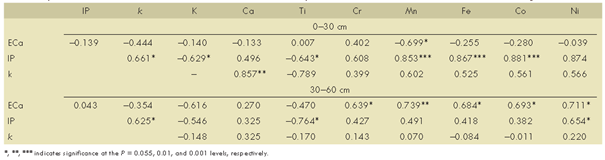
For the quadrature response (ECa), correlations were mostly nonsignificant. However, a moderate and significant (P = 0.05) correlation was measured between ECa and Mn. For the 30 to 60 cm depth interval, correlations between the different metals and ECa were generally higher. Significant correlations were observed between ECa and Cr, Mn, Fe, Co, and Ni. When operated in the VDO, the EM38-MK2-1 meter is relatively insensitive to materials at the surface, and its maximum sensitivity is at a depth of about 40 cm. This depth–sensitivity may partially explain the higher correlation between ECa and the metals in the 30 to 60 cm depth interval. At the Nottingham Park site, for the 0 to 30 cm depth interval, strong and significant (P = 0.001) correlations can be observed between the IP response and the Mn, Fe, and Co contents. For the 30 to 60 cm depth interval, less significant and mostly lower correlations were obtained between the IP response and the concentration of various metals. The relative abundance of Fe in the Chrome and Glenelg soils and the relatively high correlation between IP response and this metal support the premise that the soils and underlying ultramafic rocks contain significant amounts of ferromagnetic and/or paramagnetic minerals that affect the response of EMI sensors.
For the samples obtained from the upper 30 cm at the Nottingham Park site, significant (P = 0.01) correlations exist between k and the concentration of Ca. Nonsignificant correlations were obtained between k and the concentrations of the other metals. For samples collected from the 30 to 60 cm depth interval, correlations between k and the concentrations of the measured metals were nonsignificant.
Table 4 lists the correlations between EMI and pXRF data at the Cochranville site. For the 0 to 30 cm depth interval, strong, negative, and significant (P = 0.01) correlations were obtained between ECa and the Mn and Fe contents; however, this relationship cannot be explained at this time. For the 30 to 60 cm depth interval, correlations were mostly nonsignificant between ECa and the eight most abundant metals at the Cochranville site.
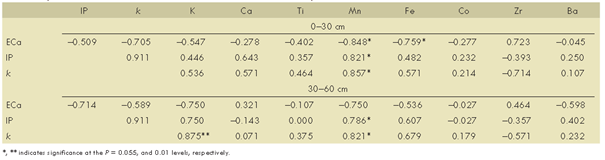
For both depth intervals, a significant correlation (P = 0.05) was obtained only between the IP response and Mn content. Compared with those at the Nottingham Park site, correlations were lower and nonsignificant between the IP response and Fe. Factors responsible for the lower and nonsignificant correlations may include lower Fe concentrations in the samples, effects of other physical parameters, and human-controlled variables related to differences in land management on the EMI response at the Cochranville site.
At the Cochranville site, significant correlations were obtained between k and the concentrations of Mn and K/Mn in the 0 to 30 cm and 30 to 60 cm depth intervals, respectively.
Conclusion
This study determined and associated the concentrations of different metals in soils formed over different lithologies in the Northern Piedmont of southeastern Pennsylvania with the responses of an EMI meter. The IP and QP responses of an EM38- MK2-1 meter and the estimated k were greater and more variable at the site underlain by micaceous schist and serpentinite than those at the site underlain by micaceous schist alone. The contrast in the EMI response and k between the two sites was associated with mineralogy and lithology differences. Spatial and depth variabilities in metal concentrations were evident at each site. Noticeable differences in the concentrations of each metal were also evident between the sites. Fe was the most abundant metal at both sites. However, the average concentration of Fe for the 0 to 60 cm depth interval was about 152% higher at the site underlain by micaceous schist and serpentinite than that at the site underlain by micaceous schists alone. At the first-mentioned site, for the 0 to 30 cm depth interval, strong and significant correlations were observed between the concentration of several metals and the IP response and k. These correlations were lower and mostly nonsignificant at the site underlain by micaceous schists only. For both sites, the correlations between the various metals and ECa were mostly nonsignificant. The contrast in the EMI and pXRF data between these two sites was largely associated with differences in k, mineralogy, and lithologies.
Original Article:
Doolittle, J., Chibirka, J., Muñiz, E. and Shaw, R. (2013), Using EMI and P-XRF to Characterize the Magnetic Properties and the Concentration of Metals in Soils Formed over Different Lithologies. Soil Horizons, 54: 1-10 sh13-01-0009. https://doi.org/10.2136/sh13-01-0009
References
[1] R. L. Van Dam, J. M. H. Hendrickx, B. Harrison, B. Borchers, D. J. Norman, S. Ndur, C. Jasper, P. Niemeye, R. Nartney, D. Vega, L. Calvo, J. E. Simms, Proc. SPIE. 2004, 5415, 665.
[2] R. Dalan, in Remote Sensing – Applications, Alabama Press, Tuscaloosa, 2006.
[3] A. Tabbagh, in Seeing the Unseen—Geophysics and Landscape Archaeology, Taylor & Francis Group, London, 2009.
[4] R. E. North, J. E. Simms, SAGEEP 2007, 20, 264.
[5] Geonics Limited, EM38-MK2-1 ground conductivity meter operating manual, Geonics Ltd., Mississauga, 2009.
[6] A. Tabbagh, Archaeometry 1986, 28, 185.
[7] M. L. Crawford, W. A. Crawford, A. L. Hoersch, M. E. Wagner, in The Geology of Pennsylvania, Pennsylvania Geological Survey and Pittsburgh Geological Society, Harrisburg, 1999.
[8] J. D. Istok, M.E. Hayward, Soil Sci. Soc. Am. J. 1982, 46, 1106.
[9] J. L. Burgess, S. Lev, C. M. Swan, K. Szlavecz, Northeastern Naturalist 2009, 16, 366.
[10] M. C. Rabenhorst, J.E. Foss, Soil Sci. Soc. Am. J. 1981, 45, 1160.
Source: Preview Image: Shutterstock